Keywords: Biophysical science, High-pressure cryocooling, Protein structures and dynamics, Water dynamics at cryogenic temperature
Background

Fig.1. (Left) Goguryeo hunting scene mural, 5th century. (Right) Muybridge’s The Horse in Motion, 1878 [1].
Historically, galloping horses were illustrated incorrectly with all four legs stretched. Painters must have believed that it was the right way to portray the motion. However, by the late 19th century, the capture of intermediate motion from a camera completely changed the general perception of galloping horses (Fig.1). As can be seen from the galloping horse example, it is obvious that intermediate states are essential to understand the mechanism correctly.
Here, we introduce a method to capture the intermediate state, called high-pressure cryocooling (Fig.2) [2]. The method is largely divided into two steps: 1) pressurization process in which different gases can be used depending on the sample and 2) rapid cooling process in which dynamics of a pressurized sample are slowed down or entrapped. Let’s see an example of how it was applied in an experiment done by our lab’s principal investigator. There is an enzyme called carbonic anhydrase that is known to be the fastest in the world having a maximum turnover rate for CO2 hydration of more than 106 s-1. Due to the extremely fast reaction rate, the process of chemical catalysis has long been considered impossible to observe experimentally. However, high-pressure cryocooling made it possible [2]. By pressurizing CO2 gas and rapid cryocooling, intermediate states were induced and captured, and it has enabled a sophisticated understanding of carbonic anhydrase’s mechanism.
This is one of the experiments conducted in the past, and more extensive experiments are currently being conducted at UNIST. The following is an introduction to the research topics that our laboratory is investigating currently.

Fig.2. (Left) High-pressure cryocooling machine installed in our lab. (Right) Simplified diagram of high-pressure cryocooling process. (a) Pressurization of sample. (b) LN2 (77K) cooling under pressure. (c) Pressure releasing process.

Fig.3. (Left) The overall shape of human carbonic anhydrase II. (Right) A closer view on the active site (Zn, CO2 binding site, and His64). Captured CO2 electron density is clearly visible [3].
Research
Biophysical study on protein dynamics: human carbonic anhydrase II (CAII)
1) Protein intermediate state analysis using high-pressure cryocooling

Fig.4. The active site of CA II at different internal CO2 pressures. (a) 15 atm CO2 CA II, (b) 7.0 atm CO2 CA II, (c) 2.5 atm CO2 CA II, (d)15atm CO2 CA II – 50s, (e) 15 atm CO2 CA II – 1h [3].
Enzymes are biological catalysts that speed up chemical reactions in living organisms and their biochemical reactions occur in the elaborately structured microenvironment within the active-site. Since the actions of enzymes that occur in sequence are closely related to their highly optimized active-site, it is extremely difficult to reveal the mechanism without structural information of intermediate states. As explained in the background session, high-pressure cryocooling technique can be used to capture the ultra-fast dynamics of enzymes and the target enzyme is human carbonic anhydrase II (CA II). Specifically, we are attempting to understand the inner mechanism through the following topics: 1) water network restoration mechanism [3]. 2) revealing the relationship between structural modifications and functional changes [4]. 3) the catalytic roles of metal ions in a metalloenzyme system [5].
2) Photolysis-induced protein crystallography
Additional methods also provide deep insight into CA II activity. The development of X-ray free-electron laser (XFEL) has enabled time-resolved serial femtosecond crystallography experiments. After the pump laser hits the sample to initiate a reaction by photolysis of the caged compound, the XFEL beam hits the sample to collect X-ray diffraction for crystal structure analysis. We have already demonstrated the feasibility of pump-probe time-resolved SFX experiments to collect intermediate structures of CA II at the XFEL beamline [6] and we are in the progress of visualizing the pathways of substrate/product entry/exit from the active site.
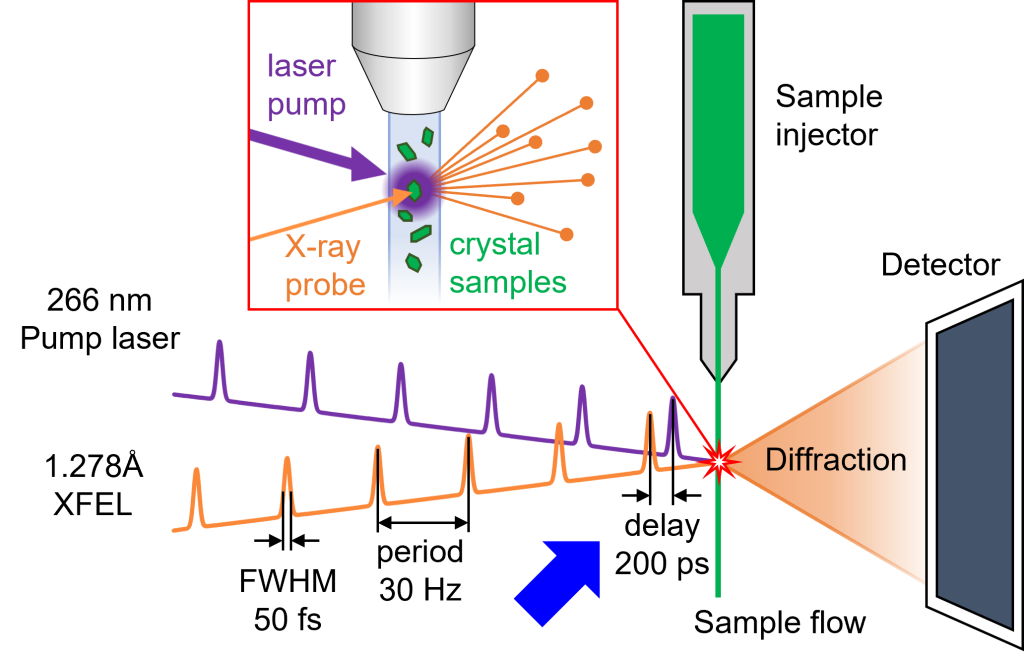
Fig.5. General schematic of protein time-resolved serial femtosecond crystallography using pump-probe method [7].
Water dynamics at cryogenic temperatures
One of the well-known surprising anomalous properties of water is that it is still unknown why water has the maximum density at 4°C. To reveal liquid water’s anomalous behavior that cannot be explained by the simple chemical structure, polyamorphism has been proposed, which refers to the ability of water to exist in various amorphous forms. However, the preparation of amorphous ice is not simple because the water molecules must be rapidly cooled to below freezing temperatures to prevent crystallization. As a breakthrough, high-pressure cryocooling has enabled the generation of high-density amorphous ice (HDA) and low-density amorphous ice (LDA) at cryogenic temperatures. The prepared samples of amorphous ices are combined with various research methods to uncover the secrets of water.
1) Liquid-liquid phase transition at cryogenic temperature

Fig.6. Correlation between crack healing and phase transitions of LDA and HDA states upon warming. (Up) The beginning of crack healing within the 0.9 M NaK tartrate solution prepared in the HDA state is shifted to a lower temperature, 120 K. (Down) Crack healing within the 1.5 M NaCl solution prepared in the HDA state is also observed around 120 K [8].
Rapid crystallization has prohibited the observation of the hypothesized glass-to-liquid transitions between the high-density amorphous (HDA) and low-density amorphous (LDA) water states. The crack healing method combined with high-pressure cryocooling made it possible to observe the glass-to-liquid transition. When HDA ice changes to the LDA state at temperatures as low as 120 K, crack healing, a sign of liquid, takes place. These findings support theories that LDA and HDA ice are thermodynamically distinct and that they are continuously connected to two different liquid states of water.
2) Solvation dynamics: cryo-photo physics

Fig.7. Scheme for solvation dynamics
It is still unclear how amorphous ices behave at low temperatures. High-pressure cryocooling can be combined with solvation dynamics using fluorophores to analyze amorphous ice. Photoexcited fluorophores change the orientation of water molecules, and local environment information is delivered through fluorescence emission. As a result, this concept may provide insights into the properties of amorphous ice using spectroscopic approaches.
References
[1] Papacosta, P. (2018). Muybridge, the Galloping Horse and revealed Errors in Art. International Journal of Art and Art History, 6(1). https://doi.org/10.15640/ijaah.v6n1a2
[2] Kim, C. U., Kapfer, R., & Gruner, S. M. (2005, June 24). High-pressure cooling of protein crystals without cryoprotectants. Acta Crystallographica Section D Biological Crystallography, 61(7), 881–890. https://doi.org/10.1107/s090744490500836x
[3] Kim, J. K., Lomelino, C. L., Avvaru, B. S., Mahon, B. P., McKenna, R., Park, S., & Kim, C. U. (2018, January 1). Active-site solvent replenishment observed during human carbonic anhydrase II catalysis. IUCrJ, 5(1), 93–102. https://doi.org/10.1107/s2052252517017626
[4] Kim, J. K., Lee, C., Lim, S. W., Andring, J. T., Adhikari, A., McKenna, R., & Kim, C. U. (2020, September 9). Structural insights into the effect of active-site mutation on the catalytic mechanism of carbonic anhydrase. IUCrJ, 7(6), 985–994. https://doi.org/10.1107/s2052252520011008
[5] Kim, J. K., Lee, C., Lim, S. W., Adhikari, A., Andring, J. T., McKenna, R., Ghim, C. M., & Kim, C. U. (2020, September 11). Elucidating the role of metal ions in carbonic anhydrase catalysis. Nature Communications, 11(1). https://doi.org/10.1038/s41467-020-18425-5
[6] Lomelino, C. L., Kim, J. K., Lee, C., Lim, S. W., Andring, J. T., Mahon, B. P., Chung, M., Kim, C. U., & McKenna, R. (2018, May 17). Carbonic anhydrase II microcrystals suitable for XFEL studies. Acta Crystallographica Section F Structural Biology Communications, 74(6), 327–330. https://doi.org/10.1107/s2053230x18006118
[7] Kim, J. K. (2022, May 18). Structural Study on Intermediate States of Carbonic Anhydrase Using X-Ray Crystallography.
[8] Kim, C. U., Tate, M. W., & Gruner, S. M. (2015, September 8). Glass-to-cryogenic-liquid transitions in aqueous solutions suggested by crack healing. Proceedings of the National Academy of Sciences, 112(38), 11765–11770. https://doi.org/10.1073/pnas.1510256112